
Photo by: Sauber F1 Team
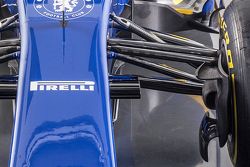
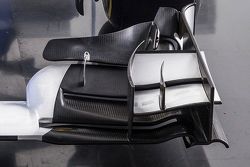
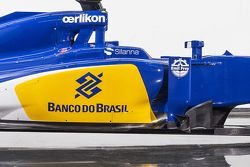
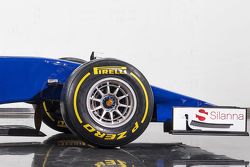
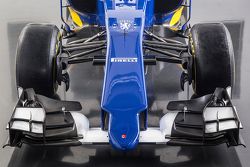
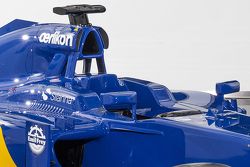
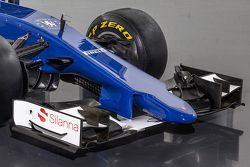
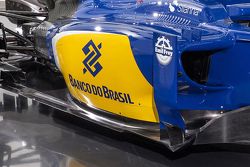
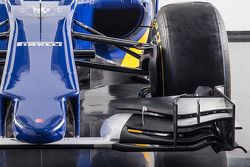
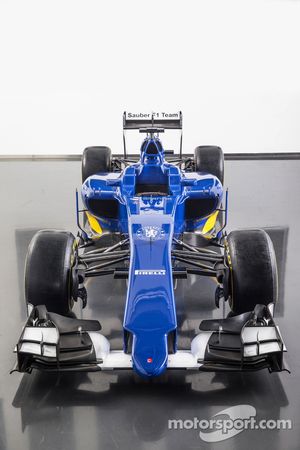
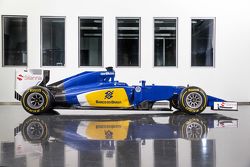
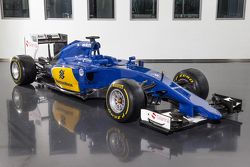
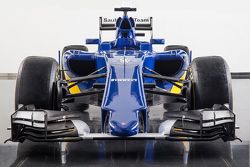
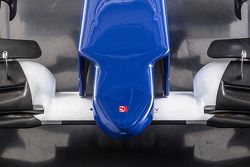
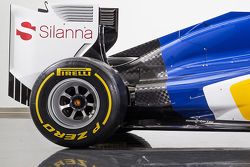
Monocoque
The monocoque constitutes the core of every Formula One car. It is the driver’s workplace and survival cell in one. The engine is flanged onto it at the rear, the car’s nose at the front. The shape of the monocoque is dictated by various factors. These include the dimensions – as per regulations – of the cockpit opening, for example, as well as the length of the wheelbase, the size of the fuel tank, the driver’s measurements and various aerodynamic requirements.
In the design process for the monocoque, the first stage is to define the surface form. Following that, finite-element calculations are carried out to ensure that the safety cell complies with the necessary levels of rigidity and strength identified by the engineers. These are based on the one hand on the dynamic loads experienced by the car, and on the other on the safety stipulations of the FIA. Standards have become increasingly stringent over recent years, ensuring a significant increase in passive safety for the drivers. The most important tests are the frontal crash (with the nose section) at a speed of 15 m/s, the stationary load test for the rollover bar, which has to withstand around 12 tonnes of pressure and the newly introduced side impact structure squeeze test, where ten and 15 tonnes are applied simultaneously to the upper and lower side structures to test the monocoque’s strength. A total of four dynamic and more than a dozen stationary tests are carried out on the car as a whole.
The monocoque consists of a carbon-fibre/aluminium honeycomb composite, which makes for extremely high rigidity and strength while keeping weight low. The composite engineers work out how many layers of carbon fibre are needed in which areas of the car so as to fulfil the diverse requirements. Here they can also select various types of carbon fibre, depending on whether forces are exerted from a single direction or several. In areas subjected to particularly high loads, up to 60 layers of carbon fibre may be stacked on top of each other. In total, a monocoque is made up of some 1,500 individual carbon-fibre elements.
It consists of two half-shells into which additional strengthening elements are glued. Following several curing stages in the autoclave, the two halves are glued together. The final stage involves the assembly of numerous securing components.
Their extremely high strength means that monocoques provide drivers with maximum protection even in major accidents. Because the fuel tank is also contained within the monocoque, dramatic accidents involving fires are a thing of the past. After a crash, the safety cell can almost always be repaired.
At Hinwil, four monocoques are built per year for use in races and testing as well as for rig tests. Every single safety cell has to be homologated by the FIA, although only the first example has to pass the full range of tests.
Carbon fiber
With the exception of the engine, gearbox components and wheel rims, a Formula One car is made almost entirely of carbon fibre. The salient properties of carbon are its high rigidity and strength coupled with very low weight. It compares with steel in terms of rigidity, yet it is around five times lighter. The downside is the highly complex manufacturing process and the high cost of the material: one square metre of pre-impregnated carbon-fibre sheeting costs from 50 to 200 euros.
Carbon fibres have a diameter of five to eight micrometres. Generally, between 1,000 and some 20,000 fibres are bundled together before being woven into fabric-like structures.
Approximately 20 different types of carbon-fibre material are used in Formula One, largely distinguished by their structure and the type of resin with which they are impregnated. If forces only come from one direction, a unidirectional weave is used, whereas forces emanating from various directions require a bidirectional weave. Specialist composite engineers determine which weave is required with which resin and how many layers are needed to achieve the desired properties.
The manufacturing process for carbon-fibre parts involves several stages. First the component is designed on the computer by means of CAD (Computer Aided Design). This data is then refined and serves as a basis for CAM, or Computer Aided Manufacturing. Using a five-axis milling machine, the form is cut into a tooling block that serves as a positive mould. The laminators lay the pre-shaped carbon-fibre pieces on this tooling block. Once this process has been completed, the entire item is packed into a polythene bag, vacuum-sealed and placed inside an autoclave, where it is cured for ten to 20 hours at a temperature of around 50°C. Following some final touches, the resulting negative mould is then ready to be used for the manufacture of the actual carbon-fibre component.
The laminators lay the pre-shaped carbon-fibre pieces on top of and alongside each other in the negative mould following plans drawn up by the composite engineers. Depending on the component, these can number up to several hundred. When everything is ready, the mould with its carbon-fibre inlay is likewise packed into a polythene bag, vacuum-sealed and baked for five or six hours at a temperature of approx. 150°C. After this curing process, the individual parts are further refined and combined to form complete components. A front wing, for example, consists of around 20 individual carbon-fibre parts. For components that have to be exceptionally robust, Kevlar or Zylon are used alongside the carbon fibre.
Brakes
As a rule, it is not so much the speed as the deceleration that really takes a rookie driver by surprise the first time he gets behind the wheel of a Formula One car. The braking power – which can sometimes peak at over 5g – is quite literally breathtaking.
This kind of deceleration derives from a combination of high aerodynamic downforces and extremely high-performance braking systems. Unlike road cars with their steel brake discs, racing cars use carbon-fibre discs and pads. Not only are these components much lighter than their steel counterparts – a complete set of discs and pads weighs less than 10 kg – but they can also generate vast amounts of braking energy.
While carbon fibre is used for the discs and pads, the front and rear callipers are made of aluminium alloy. On safety grounds, Formula One cars have two brake circuits, one front and one rear,
and therefore also two master cylinders – one for each circuit. The diameter of the master cylinder is variable, depending on the preferences of the driver and the stipulation since 2014 that their master cylinder diameters are within two millimetres of each other. A larger-diameter master cylinder limits maximum braking pressure but will give better feel. The driver’s foot has to pile on plenty of pressure too – coming into the first chicane at Monza, drivers will typically apply anything up to 150 kg of force at the pedal. But they can be sure of getting results: cars need no more than 2.5 seconds and 130 metres to brake from 335 km/h to 90 km/h. For the driver, the effect is like being punched in the pit of the stomach.
For maximum deceleration, it is important to keep the brake discs at the right temperature. The optimal range lies between 350° and 550° Celsius, although short peaks of up to 1,000° are permissible. Different race circuits make different demands in terms of brake venting. A track like Montreal, for example, where the brakes take a lot of punishment, will require a high degree of brake cooling – unlike Silverstone, where the demand is low.
When following the safety car, the temperature of the brakes can soon fall below 200°C. This is always a challenge for the drivers, who have to increase the temperature of their brakes as much as possible just before the safety car is withdrawn and the race restarts. But they have to be careful, otherwise there is a risk the brake discs could vitrify. In other words, the surface of the discs could harden, resulting in significant loss of braking performance.
Finally, it is perfectly normal practice for Formula One drivers to alter the brake force distribution on their car during the race. In the past this was performed by using a lever in the cockpit, however, since 2014 a regulation change associated with the new power unit permits the use of a brake-by-wire (BBW) system, which regulates the pressure to the rear circuit. This enables the driver to modify the brake balance of the car via a switch on the steering wheel. If the front wheels are showing a tendency to lock, the driver can direct more brake force to the rear in order to reduce the load on the front wheels. Drivers also have a second switch with which they can adjust the brake balance shape to be suitable for individual corners.
Seat
As well as generating immense deceleration under braking, Formula One cars also subject the drivers to over 4 g of lateral acceleration through corners. During a race these forces are repeatedly exerted on the drivers over a period of one-and-a-half to two hours, which means the perfect seating position is absolutely crucial as even the tiniest pressure points can lead to pain or cramp. That is why each driver uses his own seat that has been precisely tailored to his body measurements.
When a new seat is manufactured, a basic carbon-fibre shell is lined with a polythene bag. This contains either a dual-component foam or polystyrene granules which are then vacuumised. The driver gets inside the seat and waits until the mass gradually moulds itself to his body shape. While this is going on, small modifications are repeatedly carried out. In addition, the position of the steering wheel and the pedals are adjusted. When the perfect fit has been established, the seat foam or polystyrene granules are left to harden.
This kind of seat fitting will occupy a driver for between a half and a full day. The resulting seat is a transitional version which will be used for the initial tests and serve as a prototype for the permanent seat. To create the definitive seat, the interior surface of the provisional model is electronically scanned. The engineers then use this scan to create a mathematical surface based on which the shape of the seat is milled into a tooling block. Through the layering of individual carbon-fibre sheets, the final seat takes shape before being cured in the autoclave.
In the final stage the seat is given its finish, which includes cutting the apertures for the safety and rescue belts and adding a layer of padding roughly one millimetre thick. A finished seat weighs in at around three kilograms.
Steering wheel
The steering wheel of a Formula One car acts as the driver’s command centre. He uses it to steer, operate the clutch and change gear, as well as controlling numerous electronic functions by means of various buttons and switches.
The first stage of the design process involves the engineers specifying the functions that are to be controlled by buttons or rotary switches. Following that, the initial layout is determined before a provisional version of the steering wheel is made by means of rapid prototyping. Now the driver can judge whether all the controls are in just the right position. If not, he advises on where he would like changes to be made.
Manufacture of the definitive wheel can now start. A carbon-fibre shell with a lid forms the basis. The holes for the switches and buttons are drilled into the lid before the foam for the steering wheel grip is applied, which is in turn wrapped in carbon fibre. During the finishing process, various coverings are used. Depending on driver preference, the grip can be lined with leather or even with a silicon mass moulded to the shape of the driver’s hands.
Now the buttons and switches are mounted and wired up to the circuit board before the display is connected as well. Since 2008 the circuit board and the display have been part of the SECU (Standard Electronic Control Unit) and are available from the FIA as standardised components.
Once all the electronics work has been carried out, the specialists start mounting the mechanical parts on the reverse of the steering wheel; these include the gearshift and clutch paddles as well as the quick release mechanism. It is a familiar sight to see drivers removing the steering wheel to get in and out of the car and then replacing it afterwards. The quick release mechanism must also pass an FIA test in which the driver has to be able to vacate the cockpit within five seconds.
Before the steering wheel is deployed on the track, it has to successfully pass an FIA crash test, which simulates the impact of a driver’s helmet. Once the tests have been successfully completed, the buttons and switches are glued onto the reverse of the frame – and the 1.8 kg high-tech component is ready for action.
Sauber F1 Team
Be part of Motorsport community
Join the conversationShare Or Save This Story
Subscribe and access Motorsport.com with your ad-blocker.
From Formula 1 to MotoGP we report straight from the paddock because we love our sport, just like you. In order to keep delivering our expert journalism, our website uses advertising. Still, we want to give you the opportunity to enjoy an ad-free and tracker-free website and to continue using your adblocker.
Top Comments